At the Tesla Autonomy Event in April, Elon Musk said the Disruptors of Detroit were working on a new battery pack that would last a cool million miles, and said it would be available next year. Now Tesla battery research partner Jeff Dahn and his team have released a paper in which they describe this million-mile battery cell.
The new Li-ion battery cell features a next-generation “single crystal” NMC cathode and a new type of electrolyte. Dahn’s team has extensively tested the cells, and believe they could enable a battery pack that lasts over a million miles in an EV.
The team’s paper, A Wide Range of Testing Results on an Excellent Lithium-Ion Cell Chemistry to be used as Benchmarks for New Battery Technologies, was published in the Journal of The Electrochemical Society. The following brief excerpt (via Electrek) describes the results of testing the new cells:
“Up to three years of testing has been completed for some of the tests. Tests include long-term charge-discharge cycling at 20, 40 and 55° C, long-term storage at 20, 40 and 55° C, and high precision coulometry at 40° C. Several different electrolytes are considered in this LiNi0.5Mn0.3Co0.2O2/graphite chemistry, including those that can promote fast charging. The reasons for cell performance degradation and impedance growth are examined using several methods. We conclude that cells of this type should be able to power an electric vehicle for over 1.6 million kilometers (1 million miles) and last at least two decades in grid energy storage.”
This is a huge advance – the new cells last two to three times longer than Tesla’s current cells – and if the company can bring the new technology into production in a reasonable timeframe, it could radically change the economics of EVs.
The paper notes the importance of long-lasting batteries for such vehicles as robotaxis, long-haul trucks and transit buses. In these applications, a battery’s ability to deliver a high number of charge/discharge cycles is critical, in contrast to the consumer vehicle market, in which maximum range is the most important feature (at least from a marketing standpoint).
The paper also mentions vehicle-to-grid applications, which could someday allow EV owners to earn revenue from their cars while they aren’t being driven (see the upcoming issue of Charged for a profile of Fermata Energy, a pioneer in this space).
Meanwhile, job listings on Tesla’s web site seem to confirm rumors that the company plans to start manufacturing its own battery cells (as reported by Electrek).
The possibilities are endless.
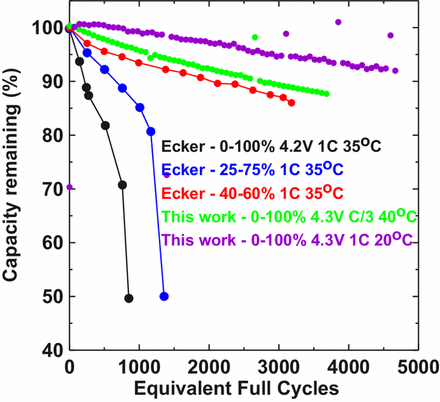
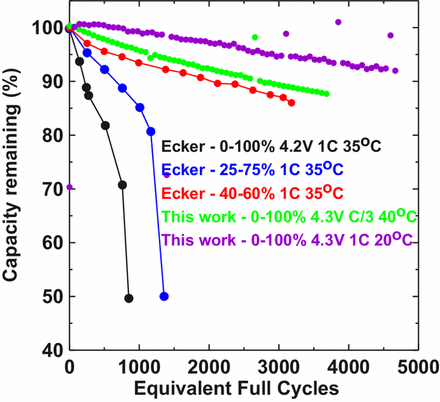
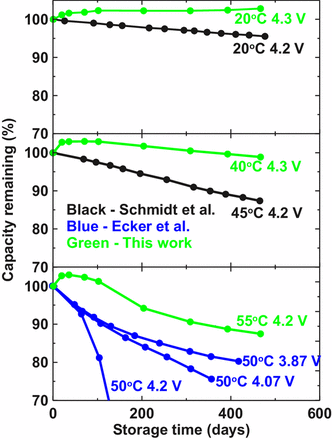
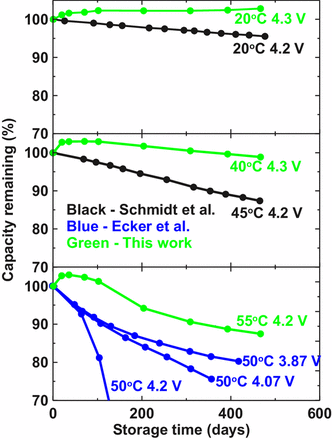
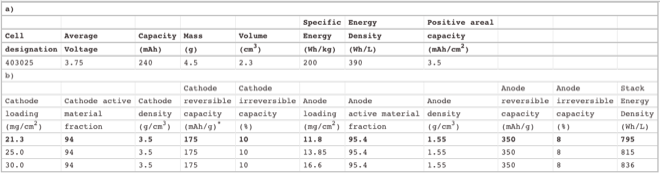
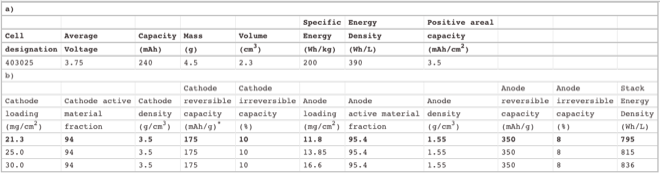
Source: Journal of The Electrochemical Society via Electrek