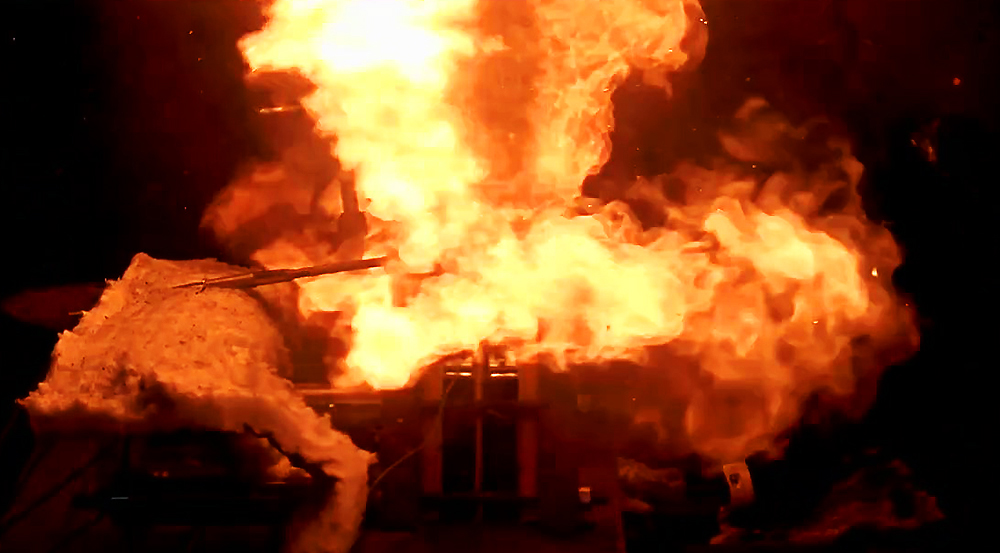
Thermal runaway propagation is a complex challenge. No matter the cell chemistry or pack architecture, lithium-ion batteries are at risk of experiencing a thermal runaway event. Although there is no one-size-fits-all solution, battery engineers do not need to reinvent the wheel when developing a solution to meet critical safety requirements. By understanding the intricacies of each stage of thermal runaway, engineers can discover which levers to pull to achieve their performance and safety goals.
What is Thermal Runaway?
Lithium-ion batteries generate a small amount of heat as they cycle. The chemical reactions within the cells can speed up when triggered by mechanical (e.g., punctured cell), electrical (e.g., overcharging), and thermal abuse (e.g., overheating). As the temperature increases, the cell loses the ability to dissipate heat, which can result in the cell catching fire (i.e., thermal runaway). Thermal runaway in a single cell is a maintenance event but can quickly propagate to other cells — thermal propagation – which is a safety issue.
Thermal Propagation Over Short and Long Timescales
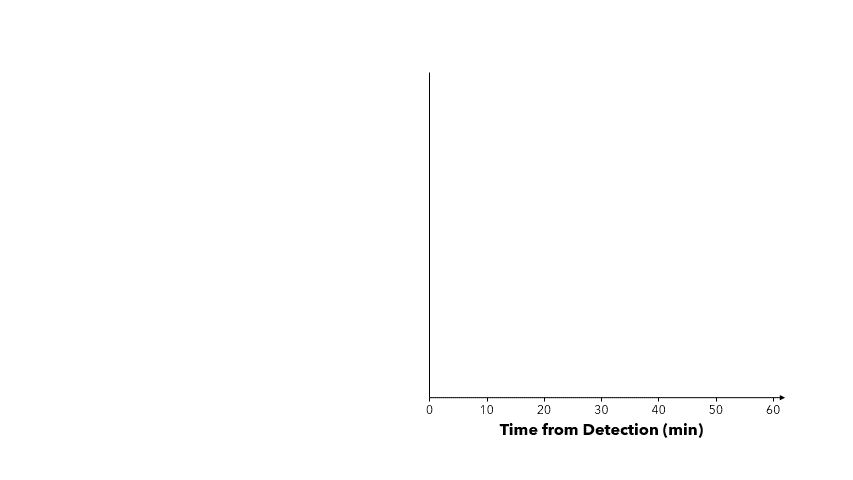
Current guidelines recommend that all passengers must be able to safely exit the vehicle within the first five minutes of a thermal runaway event being detected. As battery engineers better understand the technology, more rigorous regulations and increased timetables are likely on the horizon. The ultimate goal is to create non-propagating systems, but the industry has a long way to go.
Shorter-Term
The first few minutes of a thermal runaway event are explosive and violent. Understanding these mechanisms is crucial to mitigate thermal propagation to meet five-minute regulations.
1. Cell-to-Cell Conduction
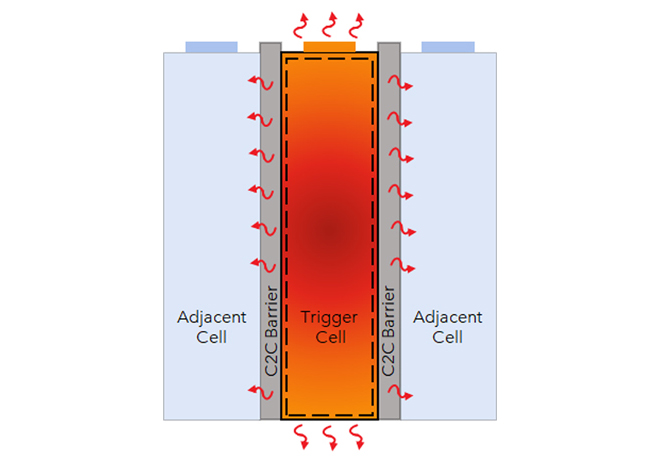
Cell conduction occurs when energy from the trigger cell transfers to an adjacent cell via face-to-face contact. The heat developed within pouch and prismatic cells is shared with the two adjacent cells, while cylindrical cells will generally share with six neighbors (edge/corner effects notwithstanding).
An effective cell-to-cell (C2C) barrier is the first line of defense to combat thermal propagation, serving as a firewall between adjacent cells. It should be a conductive barrier, insulator, or firewall between cells. During thermal runaway, a C2C barrier’s role is to protect adjacent cells from the trigger cell. It should be as thin as possible, provide exceptionally high thermal resistance while compressed, and resist temperatures of 850°C or higher.
PyroThin is an industry-leading cell-to-cell barrier because it provides thermal and mechanical protection over a vehicle’s entire lifecycle in an ultrathin, lightweight format. Aspen’s unique aerogel technology enables PyroThin to act as both compression pad and fire barrier. PyroThin is a tunable platform that can be customized to meet thermal, compression response, and thickness requirements.
2. Primary Combustion
Gas management is key to making a non-propagating design. The ejection of gas from a cell occurs in two steps:
- During primary combustion all the reactants for the combustion process come from inside the cell. Primary combustion typically begins inside the cell and can then transition outside as the reactants are ejected.
- Secondary combustion occurs when the hot, fuel-rich materials inside the cell are ejected and react violently with air in the head space above the cells.
Gas management is critical to extending propagation delay times beyond the five-minute threshold. Unless properly controlled, hot gases can rapidly spread over the top and sides of the modules, directly triggering adjacent cells, and short-circuiting all the other protective mechanisms.
3. Hot-Particulate Ejection
In addition to releasing hot gases from a cell in runaway, there is often the high-velocity expulsion of hot particulates including molten aluminum, melted or carbonized plastic bits, and solid chunks of copper. These particulates make the problem of gas management even harder, as they subject the surrounding areas to both heat and concentrated erosive blast for as much as 60 seconds.
Longer-Term
Containing a thermal runaway event with PyroThin can be like catching a wild animal — it begs the question: “Now what?” All that thermal energy must still be drained away, and there are multiple pathways for it to run down. As described below, each pathway must be controlled, so all that contained heat does not flow into the adjacent cells.
4. Secondary Conductive Pathways Kick In
As thermal propagation events run longer, secondary conduction pathways become critical and there are a lot of them, including:
- Bus bars are often made of aluminum or copper, making them good electrical conductors. Bus bars provide a pathway from one cell tab to its neighbor. Cell-to-cell barriers have zero impact on these thermal bridges because they do not extend beyond the profile of a cell.
- A cooling plate, whether active or inactive, is generally a large sheet of aluminum. It forms a pathway that tunnels underneath a C2C barrier, bypassing it as a thermal bridge.
5. Natural Convection Across Air Gaps
Natural convection (as opposed to the pressure-driven convection during active venting) occurs when hot combustion products spreads across and around the module, directly heating the adjacent cells.
6. Active Cooling
Active cooling is a wild card because a vehicle’s thermal management system may not always be intact and functional, especially in a post-crash scenario. If available, active cooling systems can – over long enough time scales — absorb excess heat and reject it safely. Thermal management systems are generally ineffective during the initial five-minute period of a thermal runaway event because the heat generation rate overwhelms the system’s heat-rejection capacity. However, over longer timescales (e.g., 15, 30, 60-minutes), active cooling is good at removing the excess thermal energy and rejecting it, helping mitigate thermal propagation.
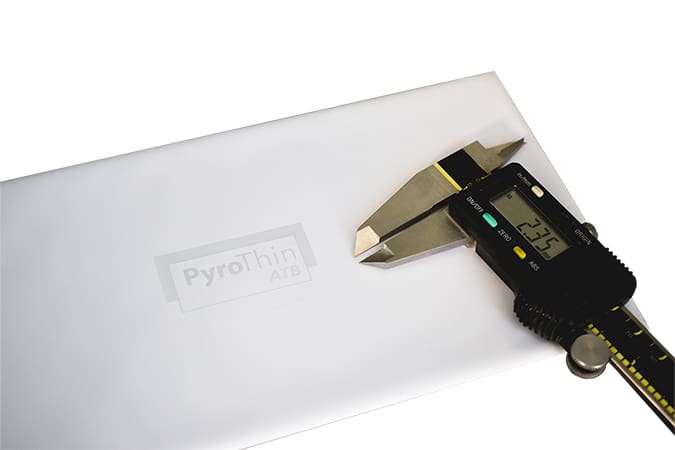
When developing a thermal propagation prevention strategy, battery engineers should keep all the above pathways in mind. In recent mini-module testing, PyroThin repeatedly stopped thermal runaway propagation on a cell-to-cell level. While only a piece of the puzzle, isolating cell-to-cell conduction and proving that thermal runaway can be stopped allows the focus to shift to other pathways.
Aspen Aerogels offers robust engineering design and prototyping support. To learn more about PyroThin or to schedule a meeting with our technical team, visit Aspen Aerogel’s website.